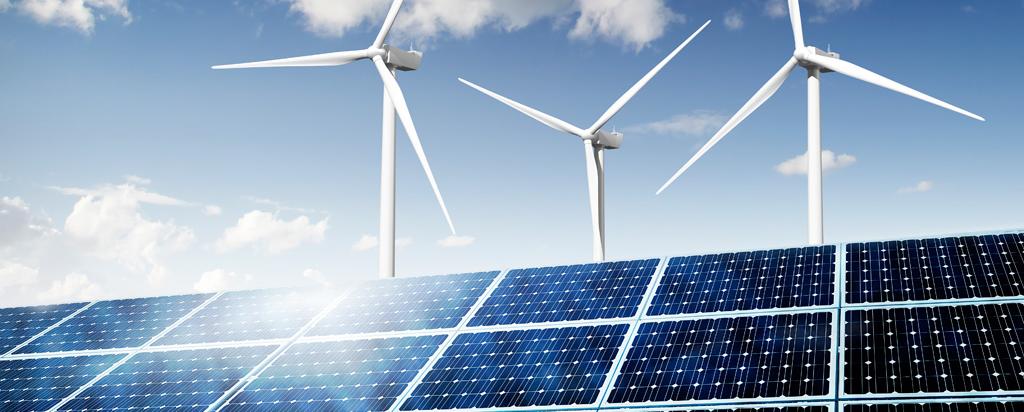
Energy Materials
Alternative energy sources, new materials, and gas sequestration technologies have risen as a result of the combined needs for energy and environmental sustainability, with the focus moving increasingly away from more traditional combustion of fossil fuels.
The Energy Materials project focuses on advancing energy technologies through the coupling of neutron scattering tools and other capabilities and expertise at ANSTO.
Neutron-scattering tools are extremely well suited for the study of functional materials for energy and excel particularly in the study of hydrogen amongst other systems.
In broad terms, the research of this project encompasses energy systems using hydrogen (hydrogen production, storage, and use in fuel-cells), solar-energy materials, and the study of energy-transport mechanisms in battery and ion-conductive materials.
Below are some examples of the areas of our research:
Hydrogen Production
One of the key challenges to the hydrogen economy of the future is to generate sufficient hydrogen to satisfy demand. Currently, H2 is sourced from hydrocarbons and coal, which negates the reduction in carbon emissions gained in using H2.
Hydrogen production from biomass and waste fuels
An alternative is to use renewable fuels such as biomass and non-recyclable wastes as a source of H2. Biomass fuels currently account for 14 % of global energy supply.
The objective of this project is to determine the optimum conditions to selectively produce hydrogen from biomass and waste fuels using a fluidised-bed gasification process coupled with in-situ carbon dioxide sequestration.
Neutron-scattering techniques will be used to monitor the hydrogen generation reactions, in-situ where possible. This will enable the elucidation of the synthesis mechanisms and ultimately identify optimum conditions for large-scale, sustainable hydrogen production.
Hydrogen production from the photoelectrocatalytic splitting of water
Semi-conducting metal oxides such as TiO2, WO3, and Fe2O3 have been intensively studied for their photocatalytic properties. While TiO2 is arguably the most studied photocatalytic material because of its stability, its activity is ultimately limited by its wide band gap, which results in a maximum absorption of only 3 % of the solar spectrum.
This research is currently not being pursued past what was achieved during the 2007-2009 period, although we anticipate continued work in this area in the future. Research has focussed previously on heteronanostructured porous thin films with complex architectures, including addressing in greater detail the electronic properties of the relatively 'simple' WO3 TiO2 multilayer thin films investigated earlier in an attempt to understand the particular activities of sandwich materials (TiO2-WO3-TiO2-Ti).
Gas-Storage Materials
Hydrogen Storage
Competitive hydrogen-powered transportation promises to transform existing industries and minimise the environmental impacts associated with fossil-fuel energy sources. The creation of new materials for the safe, efficient, and compact storage and retrieval of hydrogen is essential to the realization of hydrogen as a commercially-competitive energy carrier.
The aim of this project is to reveal fundamental insights into the interactions of hydrogen in and mechanisms of storage and retrieval from storage materials and to use the derived structure/dynamics-function relationships to create new materials with hydrogen-storage capabilities superior to existing systems. In considering methods to store hydrogen we are faced with the choice to convert it into another chemical compound from which the gas can be released (chemisorption), or to store the molecule "as is", as H2 (physisorption). This research studies both of these options.
Neutron scattering will be used to reveal insights into the physics and chemistry of hydrogen in these materials, a critical step in the design and construction of smarter and more efficient high-level hydrogen storage technologies.
Gas-Hydrates
Methane hydrates are of particular interest to the petrochemical industry as their formation can block deep-sea gas pipelines resulting in large repair costs. Research into the crystallization kinetics of methane hydration and formation, the effects of additives and inhibitors, and the atomic and molecular scale structure will be investigated using in-situ neutron diffraction. Morphology will also be investigated using small-angle X-ray and neutron scattering, including particulate morphology and agglomeration.
Fuel Cell Research
Solid-state Ionic Conductors for Solid-Oxide Fuel cells
Solid-state ionic conductors are ceramic materials through which specific ions (charged species) can move while leaving their frameworks essentially unchanged. They are critical components in solid-oxide fuel cells (SOFC's), which use hydrocarbons and other fuels more efficiently by bringing them into contact with oxide anions in a direct and controllable manner, rather than simply burning them in air.
This minimises pollution due to incomplete combustion and dramatically improves efficiency by producing electricity directly, rather than indirectly by using the heat of combustion to boil water to drive turbines to turn generators. Of particular interest is the use of fuel cells to "burn" pure hydrogen gas, with water as the only by-product.
The aim of this project is to obtain a better understanding of ionic-conduction mechanisms in materials used for SOFC's, both currently used and proposed for the future, and thereby contribute to optimising the performance of those materials and possibly discovering/developing new ones.
In-situ neutron-diffraction experiments under conditions close to those of SOFC operation (high temperature, gradient of partial oxygen pressure, atmosphere cycling) are the only way to establish a direct connection between the evolution of the crystal structure of a material with property response such as ionic conductivity and oxygen permeability.
Careful analysis of diffraction data provides additional information on sample microstructure evolution (degree of preferred orientation, crystallite size and/or microstrain) directly affecting the mechanical stability of SOFC's.
A powerful technique to investigate these materials is in-situ single-crystal neutron diffraction; however, this is rarely used because of the requirement for very large single crystals, which are difficult to grow. We will make use of the new floating-zone image furnace recently installed at the University of Sydney to grow the largest possible crystals, and investigate their structure using the unique capabilities of the quasi-Laue single-crystal neutron diffractometer KOALA to obtain structural data from crystals too small to be analysed by other neutron-diffraction instrumentation.
Investigations into the fundamentals of ionic transport are currently being pursued using model systems, such as the well-known mixed ionic-electronic conductors copper and silver chalcogenides, which have high-ionic conductivities.
Polymer Electrolyte Fuel Cell Membranes
Soft polymer membranes that can exchange hydrated protons from the anode to the cathode of a fuel cell will is developed using plasma techniques. Present membranes are expensive (~AU$1000/m2), are easily poisoned (by hydrocarbon impurities), and their ability to conduct protons is strongly coupled to the hydration, making efficient operation over 100oC quite difficult.
The polymers containing sulfonic acid groups will be developed at the Space Plasma, Power and Propulsion group at the ANU by plasma polymerization from a gaseous mixture made up of styrene and trifluoromethane sulfonic (triflic) acid monomers. The role of the triflic acid is to bring the proton conductive functions (SO3-) while the styrene monomer constitutes the skeleton element of the carbonated polymer matrix.
A dry plasma process, similar to that presently used in the microelectronics industry, has been used to deposit a low thickness membrane of a few micrometres where the morphology and chemical composition can be easily modified by changing the plasma parameters such as discharge power, gas pressures and flow rates and substrate temperature.
Using this approach it should be possible to obtain membranes with a longer lifetime, increased chemical and thermal stability, higher conductivity, and operational temperatures greater than 100oC without the need for external humidification of the reactant gases.
A number of diagnostic systems such as Langmuir probes, energy selective ion energy analysers, network analysers, optical emission spectroscopy, FTIR spectrometers and ellipsometers, will be used for thin-film diagnostics. The properties of the membranes will be characterized using scanning electron microscopy (SEM), X-ray photoelectron spectroscopy (XPS) and Fourier-transform infrared spectroscopy (FTIR).
The conductivity will be determined by electrochemical impedance spectroscopy. Measurements of methanol sorption and methanol diffusion permeability will be determined using a quartz crystal microbalance and a Hittorf diffusion cell coupled to infra-red spectroscopy.
To give new insights into the proton dynamics in the picosecond time window and in the angstrom spatial scale, a neutron-scattering investigation of the water in these films will be performed. Specifically, quasielastic neutron scattering (QENS) will reveal the average dynamics of all the protons in the film. Quantitative information from QENS on the hydrogen atom motions relative to both space and time will allow a phenomenological picture of the H dynamics in the films to be developed.
Lithium Conducting Materials for Lithium-battery Applications
Understanding the processes of lithium transfer and the changes that they cause in battery electrodes is key to elucidating the mechanisms of battery degradation. Such information is revealed using neutron-scattering experiments, enabling the development of new materials with improved cycling capacities.
Neutron-scattering tools have several unique advantages over other analyses techniques for studying lithium-conducting materials. The first is their sensitivity to lithium atoms in the crystal structure, and the second is their high penetration, allowing in-situ experiments with real commercial products instead of specially-designed electrochemical cells that only simulate battery processes.
Recent commissioning activities on WOMBAT, the high-intensity (and speed) neutron-powder diffractometer, demonstrated that neutron-powder diffraction can provide information on the evolution of phase compositions and crystal structures of electrodes during charge/discharge cycling of a lithium battery.
Semiconductor Materials for Dye- Sensitised Solar Cell Applications
Semiconductor materials form the basis of solar cells. By studying these materials in detail, the mechanisms of photocurrent generation can be understood and better materials devised.
CuSCN is a p-type semiconductor and has applications in dye-sensitized solar cell applications. Investigations of the CuSCN system have been very sparse with only limited X-ray structural data being reported. Furthermore, although CuSCN exhibits well-defined polymorphism there have been no investigations into the thermal transformations that may exist and their possible relationship to photocurrent production and solar-cell efficiency.
This project will use solid-state nuclear magnetic resonance spectroscopy, neutron and X-ray diffraction, Fourier-transform infrared spectroscopy, and computational methods (Density Functional Theory and Molecular Dynamics) to characterise the primary polymorph structures and analyse their unit-cell behaviour upon thermal transformation.
Further investigations into the structural chemistry of these systems will seek to lower the three-dimensional nature of the network structure to a one-dimensional chain by selectively functionalising the thiocyanate framework. Studies of the alteration to photocurrent densities that are induced by these changes will then be made.